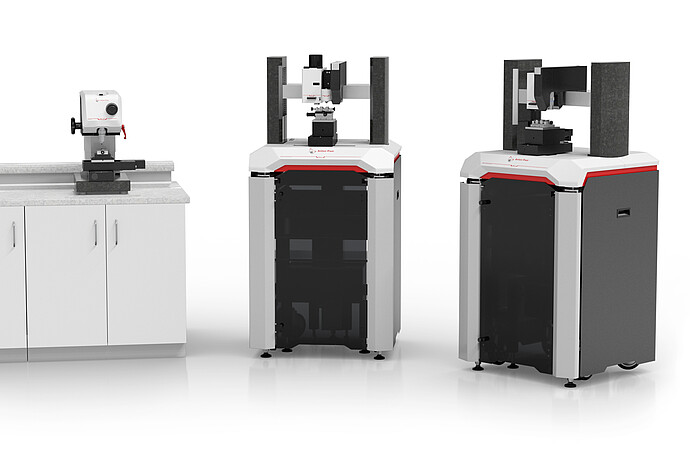
Probadores de rasgado
Soluciones del líder del mercado en pruebas de rasgado
Los dispositivos de prueba de rasgado de Anton Paar se pueden emplear para caracterizar sistemas de capa-sustrato y cuantificar parámetros, como la fuerza adhesiva y cohesiva, la fuerza de fricción, deformación y recuperación elástica utilizando numerosos métodos complementarios. Esto hace que los comprobadores de rasgado sean herramientas invaluables para la determinación de la adherencia del recubrimiento, la resistencia al rasgado y a la abrasión en la investigación, el desarrollo y el control de calidad.
Los comprobadores de rasgado pueden montarse en Step, una versátil plataforma de comprobación de superficies diseñada para alojar varios comprobadores de rayado e indentación. Cubra hasta cuatro tipos de ensayos: indentación instrumentada, rasgado, dureza Vickers automática y tribología básica.
Anton Paar Productos
Comprobador mecánico integral adaptable
Con las versátiles plataformas modulares Step, se cubre una amplia gama de fuerzas para pruebas de rasgado, pruebas de dureza convencionales e indentación instrumentada. Es posible realizar transiciones fluidas entre estos métodos sin necesidad de configuraciones adicionales. La consolidación de los 3 métodos en una sola plataforma también garantiza la adaptabilidad a futuras necesidades de medición.
Acceso a único panorama sincronizado patentado
Anton Paar es el único poseedor de las patentes de Estados Unidos 8261600 y EP 2065695. Tras el rasgado, puede grabar el panorama completo sin perder la resolución de la imagen. La imagen panorámica se muestra de manera perfectamente sincronizada con los datos de rayado. Una vez que el panorama quede grabado, podrá volver a analizar los resultados en cualquier momento.
Más información sobre la profundidad real de penetración para estudios de recuperación elástica
El sensor de desplazamiento monitorea el perfil de la superficie de la muestra antes, durante y después del rasgado (patente: US 6520004). De este modo, puede evaluar la profundidad de penetración del indentador durante y después del rayado, y obtener una visión aún más fiable de la resistencia al rayado y a la abrasión.
Control preciso de la fuerza incluso en superficies complejas
La retroalimentación de fuerza activa del sistema asegura un ensayo de rayado reproducible, incluso en caso de investigar geometrías de superficies más complejas, como muestras no paralelas, rugosas o curvas. Los dispositivos de Anton Paar son los únicos sistemas comercialmente disponibles que incorporan la retroalimentación de fuerza activa.
La fácil visualización de las muestras ahorra tiempo
Los comprobadores de rayado modulares vienen con un microscopio de doble visión que combina un objetivo de gran aumento con una cámara de visión lateral. La exclusiva función de zoom continuo le permite encontrar su área de interés a gran escala y acercarse para observar los detalles con sólo desplazarse por la gama de aumentos de 20x a 10000x.
Seminarios virtuales
Le ofrecemos una amplia y creciente selección de seminarios virtuales en vivo y grabaciones de productos, aplicaciones y temas relacionados con la ciencia.
Mostrar más